|
Anyone who has ever used a compass knows that the Earth is a giant magnet. The needle of the compass usually points towards one of two points, the magnetic poles of the Earth, located near the geographic poles. But because the compass needle is mounted horizontally, it does not show everything. Actually, the magnetic force points not just northward but also slants down into the Earth. Compass needles carefully balanced on a horizontal axis ("dip needles") point in that slanting direction, when allowed to swing in a north-south vertical plane. In fact, the angle gets steeper the closer one gets to the magnetic pole. At the pole the force is vertical. The rays of the aurora faithfully follow that slanting direction.
The Auroral Zone
That was one clue that the aurora was related to the Earth's magnetism. The other clue was found by keeping tabs on how often aurora was seen in various locations. It turned out that the important factor was distance from the magnetic pole. That pole is separated from the geographic pole, marking the Earth's rotation axis, and currently it is in the Arctic Ocean, just north of Canadian soil. The fact it is displaced towards America means Americans do not have to go as far north to see aurora as do, say, residents of Siberia, on the other side of the globe. Locations about 1500 miles from the magnetic pole are where aurora is seen most frequently: further away or nearer to the magnetic pole, they get more rare (they are quite rare at the magnetic pole itself). Fairbanks, Alaska, at the edge of the "auroral zone, " makes a good observation post.
What you usually see there are those quiet curtains and ribbons. But not always. At some times they change shape rapidly, advance, retreat or bulge out in a violent fashion, and they also get quite bright. Scientists call such an active, violent outburst an "auroral substorm, " and satellites still study the release of energy, far in space, which causes it. If you are lucky you may also see a "corona"--a burst of rays radiating in all directions. That is a caused by perspective--like the rays of the sun setting behind a cloud--and it means it the rays of the aurora are arriving right overhead.
Is the polar aurora rare? Depends on where you are! If your home is in Fairbanks, or in Tromso, Norway, or Fort Churchill, Canada--not at all. You won't see it every evening, but it is present frequently enough. In Washington D.C., or London, or Beijing, however, it is a rare event, seen only when the Sun creates "stormy conditions." On such occasions--especially near the peak of the 11-year sunspot cycle--the Sun sends out a dense cloud of hot gas, whose arrival disturbs the Earth's magnetic environment and produces a so-called "magnetic storm" (more details, further below).
Magnetic storms expand the auroral zone to locations more distant from the magnetic pole--such as Washington, London or Beijing--and also create bright auroras. If this happens on a clear night, residents in those cities can see an aurora, but it is a rare treat for them. On the right is a satellite image of an aurora extending to the "lower 48 states" of the US (note Florida, outlined by its city lights). Later that day, in March 1989, the aurora actually spread much further southward, but no satellite was in position to photograph it then. The next picture below is of a 2001 aurora seen in Purcellville, Virginia.
Electrons of the Aurora
To early observers, and well into the 20th century, the polar aurora was a great mystery. Not everything is solved even now--but thanks largely to space satellites, we have a fair understanding of the way the aurora is produced.
First question-- how high up is it? By comparing photographs taken from separated locations, an altitude of about 60 miles was found for the green aurora, and up to about twice as much for the red one. Clues like that led scientists to conclude that "something out there" was shooting towards us beams of fast electrons, somewhat like the ones painting the picture inside a TV picture tube. In a TV, electrons hit a screen, come to a stop, and their energy is converted to light. Something similar happens with the electrons that cause the aurora: they collide with atoms in the upper fringes of the atmosphere, give up their energy to those atoms and cause them to emit light.
And what are electrons? Tiny particles with negative electric charge, contained in all matter. At the center of every atom is a nucleus, containing almost all of its mass and always carrying a positive electric charge. The positive charge attracts electrons and binds them, and jointly the two types yield an ordinary atom, electrically neutral, with no excess charge of either kind. Atoms like this build up you, me, and anything we can see on Earth.
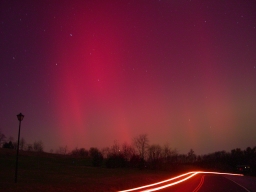 |
Red aurora over Virginia, 5 Nov.'01. Details here
|
However, sunlight can separate electrons from atoms of the gas found in space (or in the upper fringes of the atmosphere), into negative free electrons and positive "ions", atoms missing an electron or more. And because that gas is so rarefied, it can take very, very long before an electron finds a vacant nucleus and recombines again. So, free electrons are plentiful there. Still, electrons moving at 1/10 the velocity of light, like those in the aurora, need a better explanation. We will get to that, by and by.
The green and red colors are emitted by atoms of oxygen after they are hit by fast electrons. Each element emits its characteristic colors, and for rarefied oxygen, these appear to us green or red. Typically, a delay of 0.5-1 second exists between collision and the emission (in this case--not in denser surroundings!), and that is why the rays of the aurora brighten and fade so slowly. The beam of electrons which "excites" the oxygen atoms may only last a small fraction of a second, but the afterglow persists 0.5-1 seconds or more.
The Aurora and Magnetic Field Lines
And what connects the pattern of the aurora to the region of the Earth's magnetic forces--the "magnetic field" of the Earth, as that region is known? For such a region, extending far into space, a convenient method is needed to describe it there. Such a method is provided by magnetic field lines, or as they were once called, "magnetic lines of force."
Chances are you have seen a drawing of the field lines of a bar magnet. They fan out from one pole, bend around in big curves, and then converge on the other pole. The magnetic pattern near Earth is like that, too--it is as if the Earth had a small (but oh so powerful!) bar magnet in its center: the lines fan out from the region near the south magnetic pole, reach their greatest distances above the equator, then converge again near the north magnetic pole.
To define field lines more exactly, imagine you had a compass needle hanging in space, able to tell us the exact direction of the magnetic force, in 3 dimensions. Such a needle will always point in the direction of the magnetic field line at its location. North of the equator such lines converge towards the region near the north magnetic pole, just like those of a bar magnet.
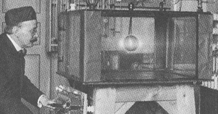 |
Birkeland and his terrella.
|
Back to the aurora. Between 1895 and 1907 the Norwegian physicist Kristian Birkeland tried to study its behavior in a lab. Inside a glass vacuum chamber he mounted a sphere with a magnet inside--he called it "terrella," Latin for "little Earth"-- and directed towards it a beam of electrons. To his surprise and gratification, the magnet steered the beam right to a patch around the magnetic poles of his small sphere, producing there, as it hit, a visible glow. He probably thought-- aha, so that is how it is done!
It turned out (and I skip a lot) that negative electrons and positive ions alike are guided in space by magnetic field lines. They tend spiral around them, meanwhile sliding along them, like beads on a wire. Because Birkeland's field lines reached the terrella near its magnetic poles, that is where his electrons came down. Similarly magnetic field lines of the Earth guide electrons of the aurora to come down in the auroral zone. No wonder the rays of the aurora pointed along such lines! Each was produced by a ray of electrons, riding its own field line down to the atmosphere. But where did those electrons start from?
The Sun's Corona and the Solar Wind
Physical processes usually require a source of energy to drive them. Think of energy as a kind of money, paying for every physical process! Any object moving at great speed needs energy to do so--its "kinetic energy"--and if the aurora contains beams of electrons moving at 1/10 the velocity of light, something must have paid the price, must have provided the energy.
Not surprisingly, it is the Sun. Indeed, why not? After all, the Sun powers almost every process on Earth: the food we eat, the coal and gasoline we burn, the winds that blow and the rain which waters the land--none would exist without the energy provided by sunlight. With the aurora, however, it is not the light of the Sun, but something more subtle, the so-called solar wind.
During a total eclipse of the Sun one can see the outermost layer of its atmosphere, the corona, a glowing halo around the darkened Sun. It turns out (by examining its light) that the corona is incredibly hot--about a million degrees centigrade, nearly 2 million Fahrenheit. Such extreme heat will tear electrons off any atom, turning the corona into a "soup" of free ions and free electrons, a strange gas known as a "plasma" which (among other things) conducts electricity. If you use fluorescent tubes, or have watched neon lights--it's the plasma inside them (not as hot as the corona) which carries their electric current and produces their light.
The plasma of the corona is far too hot for the Sun's gravity to hold it captive. Instead, it constantly expands away from the Sun and is blown off as the solar wind, filling the solar system and reaching Earth, and far beyond, past Pluto's orbit. The Earth's magnetic field, however, is an obstacle which the solar wind cannot penetrate. Like a river meeting a rock, it splits up and is diverted to flow around it. Around the Earth a cavity is formed, protected from the solar wind and known as the Earth's magnetosphere. And just as a rock in a river leaves a long shielded wake behind it, the Earth's magnetic space has a long tail on the night side--some call it the Earth's "magnetotail. "
But even though the solar wind is kept out, it manages to transmit some electric energy to the magnetosphere, by brushing against it--in particular, to the tail region. Let me say here at once, the tail is where most electrons of the aurora seem to come from, which is why in Fairbanks the brightest aurora tends to occur around midnight--even in arctic winter when the sky is dark at most other times, too.
At home, energy is carried by electric currents that flow from electric outlets to lamps, appliances and TV. The energy of the solar wind also reaches the magnetosphere (at least in part) by means of electric currents. Satellites have observed those currents near Earth: they flow in and out of the auroral zone, along magnetic field lines--for the main circuit, in on the morning side of midnight, out on the evening side, the two branches connecting (since any electric circuits must be closed!) through the high atmosphere, which (as noted) conducts electricity. One might perhaps say that we are still searching where the plug is.
 |
The US Post Office honors the Polar Aurora (2007)
|
|
Maybe the situation is not quite that dark. A lot is known. But the complete picture--where we can say "it has to be so, there exists no other way"-- that we still lack.
The Radiation Belt
 
One more detail is needed, a process called mirroring, without which the Earth might have neither aurora nor radiation belts.
Radiation belts were discovered by the first US artificial satellites, Explorer 1 and Explorer 3. In October 1957 the Soviet Union shocked the US by unexpectedly launching its two "Sputnik" satellites, while the "Vanguard," the US entry in the "space race," crashed in flames during launch, in full view of cameras. The sagging US prestige was redeemed a bit in early 1958 when instruments aboard the above satellites, designed and operated by the University of Iowa team under James Van Allen, detected a permanent belt of trapped ions, surrounding the Earth. They turned out to be protons--atomic nuclei of hydrogen atoms stripped of their single electron.
Earlier it was claimed that electrons or protons tended to be guided by magnetic field lines, like beads on a wire. The ones which guided radiation belt particles had a typical shape--they came out of Earth's southern polar region, described a wide arc across the equator, and returned back to Earth near the northern pole.
But if the analogy with beads on a wire were complete--wouldn't trapped particles slide to the ends of those wires, then hit the Earth and get lost? Quite true--except the analogy is not perfect. The ends of those lines also experience a much stronger magnetic force, being much closer to Earth than other parts, and that can be shown to repel those "beads." By being repelled from regions of strong magnetic force, trapped electrons and ions avoid reaching the atmosphere. Instead of getting absorbed, they get "mirrored" back and forth--in some cases, for years!
Electrical Currents of the Aurora
The same process is also essential to the aurora. We already mentioned large electric currents flowing from the tail into the polar regions and back again, flowing along magnetic field lines, earthward on the morning side of midnight, outbound on the evening side (for the main circuit--a secondary one also exists). They were first mapped in 1973 by two US scientists, Al Zmuda and Jim Williamson--not with a well-supported space research mission, but using a small experiment and a bummed "piggy-back" ride with a navigational satellite of the US Navy. Those currents are now known as "Birkeland currents, " honoring the Norwegian who first shot electron beams at a magnet in vacuum.
The currents along magnetic field lines in space turn out to be carried almost entirely by electrons--descending towards Earth west of midnight, rising up again east of it (being negative, their flow opposes that of the current). In light of what was noted a little earlier about the "mirror force" which repels trapped particles from regions of intense magnetic field, one may well wonder how this force affects the flow of electrons which carry those currents.
Where electrons move upwards, the mirror force is no problem--on the contrary, it helps push the electrons away from Earth, towards weaker magnetic fields. However, it is a different story where electrons come down. With radiation belt particles, the mirror force keeps them safely away from the atmosphere--but here, those electrons better reach the upper layers of the atmosphere (where the current can continue horizontally to the other branch). If not, the electric circuit remains unclosed!
So what happens? In our homes no electric currents will not flow unless a sort of electric pressure pushes them on--a pressure we call "voltage." In the home it measures 110 volt, average value (actually it fluctuates, being AC). Space currents also have a voltage pushing them, something like 40,000 volts.
In the home, if an obstacle is placed in the circuit--an electric resistance, such as a lightbulb--the voltage concentrates there to helps push the current through the bottleneck. Same thing in space! There the bottleneck is the mirror force at the ends of the field line, and to overcome it, something like 5000-15,000 volts are concentrated there, pushing those electrons through. The voltage speeds them up to about 1/10 the velocity of light, and when they hit the top of the atmosphere, they produce a bright glow. That is the polar aurora!
Substorms and slingshots
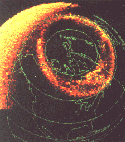 |
Aurora observed by an imaging camera aboard DE-1
|
Well--at least, one kind of aurora. Other kinds exist, too. The magnificent "ring of fire" around the magnetic pole, displayed on images from satellites (like the one here, on the left), is actually a different kind. It is too faint for the eye to see, but easily seen by spacecraft.
But the bright arcs we see from the ground are usually associated with electrical currents flowing from Earth into space--to somewhere in space. (It may be confusing, but age-old convention has it that currents flow from (+) to (-). Therefore, when negative electrons come down, the currents they carry flow upwards.) To recapitulate, the electrons must overcome the "mirror force" of the strong magnetic field near Earth, which tries to keep them out, and they do so with a concentration of voltage.
And a proof exists. The mirror force is strongest near Earth, where the intensity of the magnetic field is greatest, so one expects the voltage to be greatest there, too. And since that voltage is the source of energy of auroral electrons, they should only acquire it pretty close to Earth. Indeed, satellites show that those electrons are only speeded up near Earth, in the last 2-5000 miles before they hit.
Not all such currents are directly connected to the solar wind. A very bright and violent type of auroral arcs--associated with the "auroral substorms" mentioned before--seem to be produced (in part or totally) inside the magnetotail. In such cases the tail behaves a bit like a slingshot. The solar wind pulls its field lines and stretches them to the limit. Then, when they get released and bounce back, they create (for a while--say half an hour to an hour) strong electric currents, and many, many auroral electrons.
Those sunspots
The Sun gives out sunlight, and its hot corona produces the solar wind, the source of the aurora's energy. But the Sun is also magnetic--with a large-scale polar magnetic field (a bit like the Earth's), as well as sunspots, compact regions of concentrated magnetism. These too influence the aurora--through the interplanetary magnetic field, carried out from the Sun by the solar wind (won't go into that), and through occasional magnetic storms.
Most people have heard about sunspots--dark markings on the sun (a sunspot group is pictured on the left). Their number rises and falls with an irregular cycle of about 11 years, and some people have speculated (not too convincingly) that it matches the rise and fall of climate, the stock market, warfare and more.
That cycle was first noted by an amateur astronomer in 1843--more than two centuries after sunspots were first discovered (by Galileo and others). Astronomers had missed it completely, leaving it to Heinrich Schwabe, a German pharmacist and amateur astronomer. Schwabe searched for a planet orbiting close to the Sun inside the orbit of Mercury, tentatively named "Vulcan." You only see stars at night, so to detect one very close to the sun, you either need a total eclipse of the sun, or else you look for a dark spot crossing in front of the Sun's disk.
Schwabe looked for that spot every sunny day, and to distinguish it from sunspots (which rotate with the sun and move much more slowly), he kept tabs on sunspots as well. In 17 years he found no planet, but discovered instead that the number of sunspots rose and fell in a regular cycle.
As noted, sunspots turned out to be intensely magnetic. Associated with them are outbursts of magnetic energy, which fling fast clouds of plasma--faster than the usual solar wind--in all directions. One sign of outbursts are flares, sudden brightenings which also create a flood of x-rays. (These are not dangerous to astronauts, but the fast protons ejected at the same time can be). Another are "Coronal Mass Ejections," big bubbles of gas blown off the sun, reported in 1973 by astronauts on the space station "Skylab."
Whatever causes them, these clouds sometimes arrive near Earth and agitate its magnetosphere in a magnetic storm. The result, as noted, are bright auroras, pushed to locations much further from the poles. That is why, usually in years around the peak of the sunspot cycle, many more people get a glimpse of the polar aurora.
Artificial auroras
All the above is just a short and superficial overview of the science of the polar aurora--and I won't even try address the lore and literature. Let me conclude with two somewhat exotic aspects--artificial auroras and auroras on other planets.
Auroras, as was said, are caused by beams of electrons hitting the high atmosphere. So, if we mount an electron gun on the space shuttle or on some other spacecraft, and aim it down--can we create aurora?
In principle, yes, but in practice it's not easy to match Mother Nature. Remember, all auroras displays are at least 60 miles above our heads. It takes a lot of power to create a glow visible at that distance--especially, one as large as an auroral arc!. Experiments have been carried out--notably, the French-Soviet "Arkad" experiment above northern Russia (also some by the US), and spots of light were detected, but it took sensitive instruments to see them.
However, other more drastic ways also exist--such as exploding a nuclear bomb above the atmosphere, since a bomb produces great numbers of fast electrons. Such explosions were suggested by an unconventional Greek scientist named Nicholas Christofilos. He started out as an engineer designing elevator systems, but his real interest lay in magnetic fields and in the motion of ions and electrons in them. His interest led him to discover an important idea in the design of accelerators for nuclear research, known as "strong focusing." He wrote to Columbia University in the US, describing what he did, but scientists there did not pay enough attention to the ideas of an elevator engineer in Athens. They sent a polite reply and dropped the idea. A few years later, a Russian came up with a similar concept.
However, the US Air Force remembered Christofilos and invited him to this country. Because he was interested in the trapping of particles, Christofilos proposed creating an artificial radiation belt around Earth, by exploding a small nuclear bomb above the atmosphere.
No one suspected the existence of a permanent natural radiation belt, but some small nuclear bombs had been exploded by the US Air Force high in the atmosphere, near Hawaii. They created glows in the sky with the colors of the aurora, and people in Honolulu oohd and aahd over them--I have a dim memory that "Life" even ran a picture. So Christophilos prepared his bombs (to be flown on a rocket), while Russia prepared its Sputnik and Van Allen got ready for Explorers 1 and 3.
Sputnik was launched in October 1957, Explorer 1 was launched on the last day of January 1958, the newly discovered radiation belt was described that May, and the 3 bombs of "Project Argus" were exploded in August and September 1958, above an empty stretch of the South Atlantic. No observation broke the secrecy of the project, and no newspaper told about it at the time. But the bomb's electrons were guided by their magnetic field lines to their other end, near the Azores Islands, and produced a bright aurora which was seen by observers aboard ships deliberately stationed there. The "artificial radiation belts" from the bombs lasted about 2 weeks and were monitored by Explorer 4, built by Van Allen for that purpose.
Four years later the US Air Force decided to repeat the experiment on a grand scale west of Hawaii, using a hydrogen bomb, about 1000 times more powerful. This time the auroral electrons were guided towards Samoa--close to the equator, in a region where auroras had never been seen. But the explosion was too close to the equator, in a region where trapping was much more efficient. The radiation belt hung around for years, not weeks, and quickly destroyed 3 satellites (including a British scientific satellite NASA had just graciously launched), by degrading their solar panels and depriving them of power. The Soviet Union also exploded H-bombs in space, but the following year brought the international test ban and all such experiments ended.
Other Planets
NASA spacecraft have shown that the giant planets--Jupiter, Saturn, Uranus and Neptune--are all magnetic, and do have radiation belts. Jupiter, the biggest, outdoes them all--its "central magnet" is about 20,000 times stronger than ours. It also has auroras, photographed by the orbiting Hubble telescope (see here for a recent picture). Their origin (at least that of the stronger ones) is interesting.
As noted, Earth's aurora is associated with electric currents, and so is Jupiter's. Jupiter has 4 large moons (as big as ours or bigger), and the innermost one, Io, is a strange and hellish place, with its interior heated by tides, producing sulfur volcanoes and molten lakes. As a result, it (or the atmosphere around it) conducts electricity, and as Jupiter's magnetic environment rotates past it (just as the solar wind flows past Earth), electric currents are produced along the field lines linking it to Jupiter.
We know about those currents because Voyager 1 flew close to them in 1979 and observed the magnetic pattern they produced. And we know the observed auroras are due to those currents, because the structure of Jupiter's magnetic field has been mapped. Using that mapping to trace magnetic field lines from Io to the surface of the planet, one arrives exactly at the spots where auroras are seen.
|
|
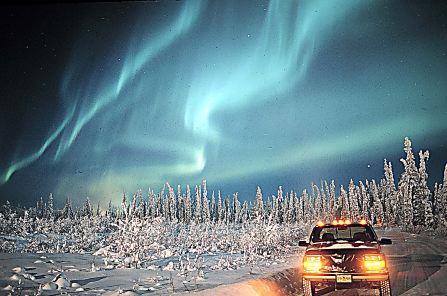 |
Aurora on 6 January 1998 (Dick Hutchinson ©)
|
|
One could go on and on, but even this quick tour should prove that the polar aurora is much more than a pretty show of lights. It also provides insight into the way physics and nature affect the space region around Earth.
Home page
Auroras Galore
The opening and closing shots of the aurora here were made by Dick Hutchinson. Dick lives in Circle, Alaska, on the Yukon river north-east of Fairbanks, in prime aurora territory. He enjoys photographing the aurora, and his collection of auroral images can give you a better feeling of "what the aurora looks like" than anything else I have seen on the web.
Other sites with auroral images:
|
|
Back to the index Page of "Exploration of the Earth's Magnetosphere"
Author and Curator: Dr. David P. Stern
Mail to Dr.Stern: education("at" symbol)phy6.org .
Created 14 November 2002
Re-formatted 10-23-2007
Above is background material for archival reference only.
|